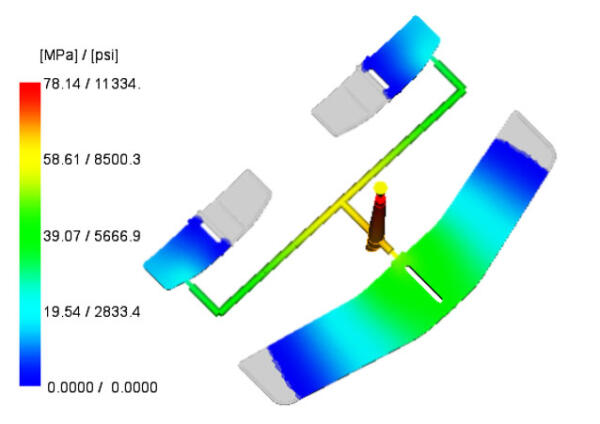
The Effect of Pressure and Temperature on Part Quality and Dimensions
Injection moulding is a complex system of machinery, fluid dynamics, and thermal conductivity. Let’s break the mould down into two simple parts, the heat exchanger and the pressure vessel, and review how they can impact overall part quality and dimensions.
Heat Exchanger
First, let’s review how the plastic makes a journey from pellet form to a liquid or a sliquid (a semi-scientific term that identifies a substance that is neither a true liquid or solid) in the injection unit.
For those unfamiliar with British Thermal Units (BTU), it represents the amount of thermal energy necessary to raise the temperature of one pound of pure liquid water by one degree Fahrenheit. To melt plastic, there’s a certain amount of BTU content that must be added to a semi-crystalline, like polypropylene, to break that structure down and turn it into an actual liquid. Breaking these crystal structures takes an enormous amount of energy in comparison to amorphous resin. Amorphous materials, like polycarbonate, technically never melt, they only get softer.
Most of us don’t think about processing plastic in BTU content, but rather we think about melt and heat deflection temperature (HDT). That is the temperature at which a polymer or plastic sample deforms under a specified load. From a thermal dynamic perspective, we only need to remove about 40% of the BTUs required to melt or soften the plastic before it is ejected from the mould. The remaining 60% of the BTU content is lost to the atmosphere during post mould cooling. We will not go into the equations necessary to understand the energy it takes to melt the plastics, but below in Figure 1, we can see the difference in cooling based solely on material selection.
When reviewing the temperature of the melted plastic in comparison to the temperature at ejection, there is an average of 62% decrease in temperature (shown in Table 1). During process development, it’s much easier to measure the melt and part ejection temperatures.
How efficiently the 40% is removed is ultimately based on where the water lines are placed, type of metal used in the mould, and the ability for the plastic part to give up its heat (referred to as thermal conductivity). In this scenario, the plastic is an insulator and is the overriding factor in achieving efficiency. The better job we do as engineers at designing the mould (shown below in Image 1), the faster the cycle time will be. In an ideal world, there would be no temperature variation across the part when it’s ejected from the mould.
However, we know in most circumstances, this is not feasible. Instead, a reasonable temperature differential of less than 20°F will yield acceptable results. In most circumstances, sinks, voids, and variation in gloss level will be detectable immediately after ejection. When the part temperature after eject is over this 20°F, there’s a likely chance you’ll end up with warp. With certain materials like Polyoxymethylene, we have to be aware that this post-mould shrinkage can sometimes take days or weeks to stabilise. Below (in Image 2), a part with thick sections shows a considerable temperature differential at ejection.
Pressure Vessel
The mould is a pressure vessel, and its influence on part quality is enormous. We are going to break this system into sections: the melt delivery system and the cavity. Since the first interaction, the plastic has with the mould is the melt delivery system, we’re going to start here.
When we look at the melt delivery system, it is important not to undersize the system to ensure there is minimal pressure loss from the nozzle machine up to the gate. In most cases, semi-crystalline needs the smallest size, followed by amorphous, and bringing up the rear would be glass or fibre filled materials of any type. At a high-level, we can start to look at the MFI (shown in Table 2) of these resins. We can see in general viscosity of each particular resin and the MFI ranges. The lower the MFI, the larger the system needs to be sized for effective filling and packing of the cavity.
When designing the melt delivery system, it’s advised to work from the part towards the moulding machine. Gates are the starting point—if undersized, it increases the filling pressure and reduces the ability to pack out the cavity. It can also increase the shear rate, which can lead to degradation of the polymer chain.
Parts with high viscosity and low MFI will likely need more than one gate to ensure the pressure loss across the cavity is not too large. Once the correct gate type, size, and quantity is determined, the journey back to the machine nozzle continues. Runner, drop, or manifold lengths should be kept as short as possible between cavities, making sure there is still adequate spacing for water lines, as previously mentioned. The higher the cavitation gets, the more difficult it becomes to manage the pressure loss.
Lastly, keeping a cold runner sprue or hot runner inlet length to under 2.000 in. is advisable. Below (in Image 3) is an example of a multi-cavity family, which exhibits vastly different flow lengths resulting in vast changes in pressure and cooling rate in the mould cavity.
We know the highest pressure in the injection moulding process is at the machine nozzle, while the lowest is at the end of fill within the mould cavity. In order to minimise defects like sink, void, warp, short shots, and dimensional instability, we as engineers have to manage the pressure loss across this entire system.
Conclusion
As we can see through these few examples, it’s critical to manage pressure loss and temperature from the moulding machine nozzle tip to the end of the cavity. Otherwise, it could result in dimensional variation and instability.
Jeremy Williams has over 19 years of experience in the plastics industry serving the medical, automotive, furniture, and appliance industries. He previously worked as a Principal Engineer, taking projects from design concept to saleable products. Jeremy earned his Master Molder II certification in 2011, became an RJG Certified Trainer in 2012, and started at RJG in 2015. In addition to his manufacturing background, he holds degrees in plastics and business. Currently, Jeremy is a Consultant/Trainer with TZERO®.
For further blog articles, visit the Know How area of RJG’s website.
RJG Technologies
+44 (0)1733 232211
Website
Email